Cytoskeleton Definition
Technically, ‘
You can relate it to our skeleton system to some extent but it doesn’t appear to be exactly the same as our skeleton system. Some parts of the cytoskeleton system act like our bones and some will work as our muscles.
The cytoskeleton is present in the cytoplasm of the cell. It extends from the nucleus to the cell membrane of the cell. The cytoskeleton is present in both eukaryotes and prokaryotes.
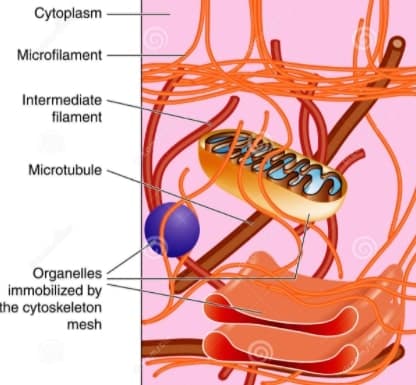
Cytoskeleton structure
Now, let’s move on to its structure,
The Cytoskeleton consists of at least three distinct structures classified on the basis of their diameter, namely,
- Microtubules,
- Intermediate filament,
- and last but not the least, Microfilaments.
Cytoskeleton Microfilaments
The cytoskeleton Microfilaments are the narrowest among these three types. It is 1-2 µm in length and 5-7 in diameter. Microfilaments of higher eukaryotes are made up of many linked monomers of a protein called actin. Because they are made up of actin monomers, they are also known as actin filament. Crosslinked actin filaments resist deformation, transmit forces, and restrict diffusion of organelles. The cortex varies in thickness from a monolayer of actin filaments in red blood cells to more than 1 µm in amoeboid cells.
The discovery of actin filament in the non-muscle cell was made in 1952 by A. Loewy. Actin subunits that come together to form a microfilament are called globular actin (G-actin). Once they are joined together they are called filamentous actin (F-actin). Each monomer of G-actin has a bound ATP, which is hydrolyzed to ADP when the monomer is added to the growing chain. Actin filament forms a double helix, 6 nm wide, with globular subunits 5.5 nm long and a 37 nm helix repeat. Like microtubules, cytoskeleton microfilaments also have polarity. Their positively charged end grows faster than their negatively charged end.
There are a number of microfilament binding proteins. Best characterized by that is myosin. Likewise muscle myosin, Non-muscle myosin has two heavy and four light chains. They
Properties of Actin filaments
The physical properties of actin filaments depend on their lengths and their interactions. At physiological concentrations, purified actin filaments are viscoelastic. Viscoelastic means that it can both resist flow, like a viscous liquid, and store mechanical energy when stretched or compressed, like a spring.
At high concentrations, actin filaments also align spontaneously into large parallel arrays called liquid crystals. Crosslinking actin filaments increases both their viscosity and stiffness.
Severing actin filaments decreases their viscoelasticity. On the other hand, shorter filaments have a higher tendency to form bundles in the presence of crosslinking proteins, so severing can actually promote the formation of rigid actin filament bundles.
Function
- Actin filaments are involved in localized cell membrane movement. A good example of this phenomenon is the leucocytes.
- Microfilament also mediates the cytoplasm streaming or cyclosis in most of the cells. Such intracellular motion helps in the migration of cell organelles at their target sites where these organelles have to perform their specific function.
- The actin filament is also involved in the provision of propulsive force. It is essential to release the acrosomal hydrolytic enzymes from the sperm into the jelly coat of the ovum. As a result, the coat is dissolved and the plasma membrane of sperm and the egg can fuse to form a zygote
Intermediate filament
These are smooth surfaced, solid and unbranched filaments. They have a diameter of 7-11 nm. It lies between those of microtubules and microfilaments. Unlike microfilament and microtubules, intermediate filaments are chemically heterogeneous in structure, composition, and solubility. T
Filament class | IF protein | Tissue distribution |
I | Keratin(acidic) | Epithelia |
II | Keratin (basic, neutral) | epithelia |
III | Desmin Peripherin Vimentin Glial fibrillary | Muscle Neurons Mesenchyme Glial cells |
IV | Lemin proteins | Nuclear envelopes |
V | Neurofilaments | Neurons |
VI | Nestin | Neuronal stem cells |
The various intermediate filament is different from each other but they show remarkable homology in their overall structure. All of them have 310 amino
Structure
The basic unit of intermediate filament is the ‘two-chain coil’ which are identical ( homopolymer) and are formed from helix interactions. On the other hand, cytokeratin is heterodimers, with one chain of one type and the other of a different type. Once the dimer has formed, it interacts with a second dimer to form a tetramer(in parallel fashion). After that, t
Now, four protofilaments make up the 10 nm diameter filament seen in cells. In all, about 25,000 individual proteins associate to form a 40µm filament( So huge to count but very small to see).
There is often a linkage between
Now, lets move on to its functions….
Function
Intermediate filaments play a significant role as motility molecules within the cytoplasm of different classes of cells.
- The keratin filaments of epithelial cells form a network around the nucleus. It also ramifies through the cytoplasm. Its role is to hold the organelles into place.
- Desmin ( in skeletal muscles) filament forms an organized network at the Z-line. They help to integrate muscle function.
- Neurofilaments play an integral part in axon transport of different macromolecules including synaptic protein towards the presynaptic membrane.
- Intermediate filaments provide the clues to the clinicians with cell markers to determine the identity of the tumor.
Microtubules
In 1800, microscopists have reported that cells and their extensions often contain a fibrous component. This was especially noticeable in cilia and flagella. In 1963, D. Slautterback coined the term microtubules. These are found virtually in all eukaryotic cells.
Microtubules are stiff, cylindrical polymers of αtubulin and β-tubulin that provide support for a variety of cellular structures. Also, Microtubules help organize the cytoplasm during interphase and form the mitotic spindle.
Structure
Microtubules are 25 nm in diameter and can grow longer than 20 µm in cells and much longer in vitro. The head-to-tail arrangement of dimers of α-tubulin and β-tubulin in the walls of microtubules gives the polymer a molecular polarity. The “plus” (β-tubulin) end grows faster than the “minus” (α-tubulin) end.
In animal cells, they usually radiate from a centrosome located near the nucleus.
The outer and inner diameter of these tubules is 30nm and 14nm respectively. Therefore, wall thickness is 8nm.
For those who don’t get wall thickness, here is some mathematics for you. It is not rocket science…….
If you don’t want to know, simply skip the mathematical part…
Here we go…
Firstly, we calculate the radius of the inner and outer ring. After that, we subtract the radius of the inner ring from the outer ring to get the wall thickness.
RO =DO/2 (RO=radius of outer ring, DO=diameter of outer ring)
=30/2
=15nm.
So, our radius of outer ring is 15. Now, let’s calculate radius of inner ring
RI=DI/2
=14/2 (RI=radius of inner ring, DI=diameter of inner ring)
7nm.
So, the wall thickness is radius of outer diameter-radius of inner diameter
15-7=8nm.
Now, let’s back to the topic
A clear, unstained zone, 5-20nm in width, often surrounds the outer wall of the microtubule. The length of microtubules vary between species and cell type. The wall of a microtubule is made up of 13 identical protofilaments. Exceptions:-crayfish nerve cord(12). These protofilaments are made up of dimers of tubulin protein.
Tubulin of microtubule
Microtubules are made up of polymers of tubulin protein subunits, which are of two types:
- α tubulin molecules
- β tubulin molecules
Dimers of tubulin have a molecular weight of 1,10,000, an alternating monomer of 55,000 molecular weight each( termed α and β).
These two polymers have a similar amino acid sequence (half of their sequences are common) but are not identical. In fact, the basic structure of this molecule and the microtubule itself is the same across the kingdoms of organisms.
The polymerization takes place in head to tail fashion so that microtubule has a definite polarity having ends as (+) plus and (-) minus destinations. This polarity helps in the assembly of the organelles.
In addition to the tubulin, several microtubule-associated proteins (MAPs) are present in different tissues. Most of the MAPs are present in brain tissue. MAP1 and MAP2 have characterized in brain tissue as the HMW( high molecular weight ) MAPs. The tubulin-binding domain of these proteins lies in the carboxyl-terminal region.
Physical Properties of microtubules
- Microtubules are cylinders constructed of longitudinally oriented protofilaments with a 4-nm longitudinal repeat arising from the tubulin subunits. Most cytoplasmic microtubules have 13 protofilaments, but microtubules in some cells have 11, 15, or 16 protofilaments.
- Microtubules are polar because all the dimers have the same longitudinal orientation. β-Tubulin is oriented toward the plus end; α-tubulin is oriented toward the minus end. The plus end grows faster and is less stable than the minus end.
- Microtubules are much stiffer than are either actin filaments or intermediate filaments. The stiffness, length, and polarity of microtubules make them valuable both for cytoskeletal support and as tracks for microtubule-based motors.
- Because they resist compression, microtubules were adapted more frequently than actin filaments or intermediate filaments to support asymmetrical cellular structures.
Function
- Microtubule provides an internal skeleton or scaffold. It provides structural support.
- It helps to maintain the position of cytoplasmic organelles.
- Serve as the primary component of the machinery responsible for mitosis and meiosis. The movement of chromosome and spindle formation during cell division is mediated by microtubules.
- They form principal elements of cilia and flagella as motile cell projections.
- They are part of the machinery that moves organelles and materials from one part to another part of the cell. A good example of this is the transport of vesicles from one membrane to another. This transport is dependent on the presence of the microtubule because the disruption of this cytoskeleton element will bring the movement to a halt.
REFERENCE
Cell and Molecular biology by Prakash S. Lohar page No. 122-130
Cell Biology:organelle structure and function by David E. Sadava chapter 9
cell biology by Thomas D. Pollard, William C. Earnshaw, 3rd edition, ELSEVIER, section IX, chapter 33-35
Very helpful notes…thanx for these notes 😊😊😊
Thank you very much for the notes they are very informative
always here to help you